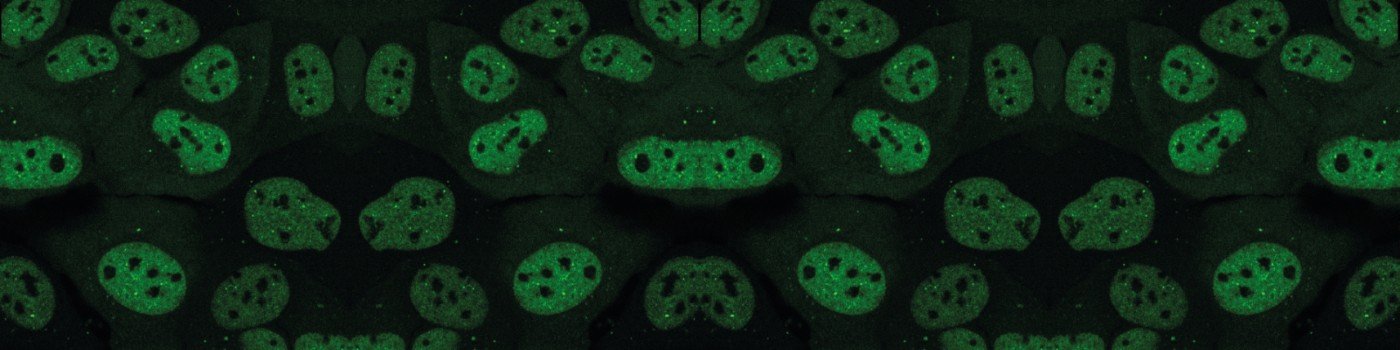
Luijsterburg lab
Transcription and DNA repair
DNA damage blocks transcription.
The timely and coordinated expression of genes is essential for life. The transcription of genes involves RNA polymerase II enzymes (RNAPII), which synthesize RNA transcripts complementary to the DNA template strand. The presence of bulky DNA damage in the transcribed strand of active genes is a major complication during transcription. Persistent stalling of RNAPII at DNA lesions is highly toxic and constitutes an efficient trigger for apoptosis. The presence of DNA lesions triggers a genome-wide transcriptional arrest due to stalling of elongating RNAPII at DNA lesions. It is essential that cells overcome this arrest and restore transcription to maintain gene expression and viability.
Transcription-coupled DNA repair.
The transcription-coupled DNA repair (TCR) pathway efficiently removes transcription-blocking DNA lesions through the sequential and cooperative recruitment of the ATP-dependent chromatin-remodeling factor CSB, the CUL4A-based (CRL4) E3 ubiquitin ligase complex containing CSA, and the UVSSA scaffold protein.
Mutations in the CSB and CSA genes cause Cockayne syndrome, which is characterized by severe developmental and neurological dysfunction. In addition to protein-protein interactions, TCR complex assembly is tightly controlled by the CRL4/CSA-dependent ubiquitylation of RNAPII at a single lysine (K1268) of the largest RPB1 subunit.
Molecular mechanism of TCR.
The concerted action of CSB, CSA, UVSSA, and RPB1-K1268 ubiquitylation facilitate the association of the TFIIH complex with DNA damage-stalled RNAPII. The subsequent association of XPA and XPG stimulate the translocase activity of TFIIH, likely resulting in TFIIH-mediated RNAPII displacement, which provides the endonucleases XPG and ERCC1-XPF access to excise the DNA lesion.
Mounting evidence suggests that RNAPII ubiquitylation activates TCR complex assembly during repair, and in parallel promotes processing and degradation of RNAPII to prevent prolonged stalling. The fate of stalled RNAPII is therefore emerging as a crucial link between TCR and associated human diseases.
Despite emerging insights, we still have large gaps in our knowledge of how TCR works and causes human disease.
The goal of our lab is to use sensitive biochemical, proteomics and genomics methods to unravel mechanisms in transcription-coupled DNA repair and its impact on human health.
Our research involves the following topics:
1. Proteomics approaches to map the TCR interactome.
To identify new proteins involved in TCR, we systematically map the interactome of repair factors using immunoprecipitation and proximity-dependent identification (Bio-ID) coupled with quantitative mass spectrometry in human cells.
2. Genetic screens to systemically map new TCR genes.
To identify the full repertoire of genes involved in TCR, we perform genome-wide CRISPR screens in collaboration with the lab of Rob Wolthuis (Amsterdam UMC). As a follow-up, we use CRISPR screening to map genetic TCR networks and their rewiring in response to DNA damage.
3. Genome-wide approaches to understand transcriptional responses and TCR.
To measure TCR kinetics throughout the genome, we used a ChIP-seq-based method called TCR-seq. We are further developing this approach together with the lab of Tomoo Ogi (Nagoya University, Japan). To understand how cells reactivate transcription after TCR has been completed, we combine ChIP-seq with nascent RNA-seq.
4. Mechanistic characterization of TCR genes and links with human disease.
To functionally dissect the role of newly identified proteins, we combine knock-out and knock-in approaches with advanced microscopy techniques, such as UV-C laser micro-irradiation and cell biological and biochemical approaches to measure DNA repair activity and transcriptional responses.